Written by Cal Orr
Even the most robust radio systems are worthless without a good, clean power supply
Technical
As seen in the August 2014 issue of Model Aviation.
A good, clean power supply is important. Our RC radios typically require a DC voltage of 4.8 to 6.0 volts. On an oscilloscope, this DC voltage would appear as a straight line, so in this case, a flat line is a good thing. An oscilloscope is a voltage meter that displays the voltage with respect to time.
In Photo 1, I have attached an old, but charged, 4.8-volt 500 mAh battery to the scope. Notice the grid on the oscilloscope’s screen. We can set the values of the divisions in the vertical direction as a voltage, and the horizontal direction as time.
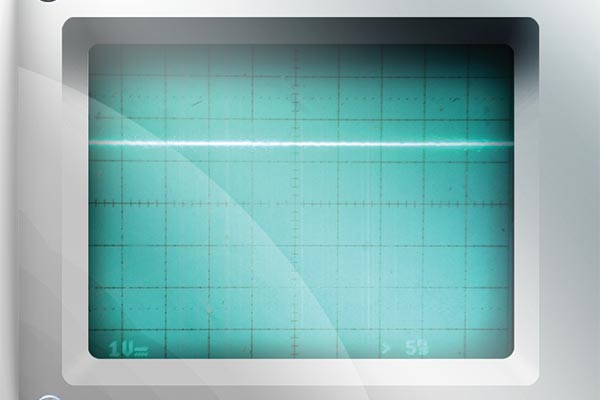
Photo 1. The oscilloscope display is set to 1 volt per division vertical and 5 milliseconds per division horizontal. The scope is connected to a 4.8-volt 500 mAh battery with no load. The trace is roughly 5.5 divisions above my ground or zero-volt line, indicating 5.5 volts.
The vertical divisions in this example are set to 1 volt per division and the horizontal divisions are set to 5 milliseconds per division. Count up from the bottom line (ground or 0 volt) to the trace. There are approximately 51/2 divisions so the scope is displaying roughly 51/2 volts. Because the trace is a straight line (time is not a factor here), we are measuring a clean DC source. What will happen if a load is put on the battery? When I attach a small lightbulb to the battery, the trace on the scope drops slightly and so does the voltage. The load of the lightbulb is a constant so the display on the scope may still look as it does in Photo 1, but slightly lower. The voltage can also be measured with a digital voltage meter, which shows 5.42 volts with the lightbulb on. This means that the battery under this small load dropped the voltage approximately 1/10 of a volt.
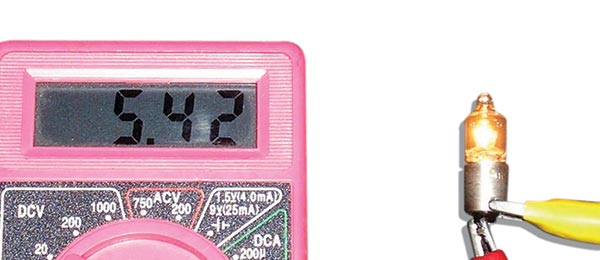
Photo 2. Digital voltage meter indicating 5.42 volts from my 4.8-volt 500 mAh battery with the load of a small lightbulb. The load dropped the voltage roughly 1/10 of a volt.
I connected the same lightbulb to another 4.8-volt battery, but with 2,700 mAh. It has the same voltage but a higher capacity. The difference between its open circuit and under load is 2/10 of a volt. You may have figured out that the larger capacity battery translates into longer flight times and less of a voltage drop under load. What happens when the load (lightbulb) turns on or off? I made an oscillator circuit that only turns the light on or off. The 1/10-volt fluctuations are visible on the scope. There is no longer a straight line. What happens when the load is no longer a small lightbulb, but a servo or several servos operating together? Photo 4 shows the scope set up the same way as before, except now the load is a receiver with four standard servos that are continually being operated with the transmitter sticks end to end. All of those voltage fluctuations are dirty power in which the radio system has to survive and operate.
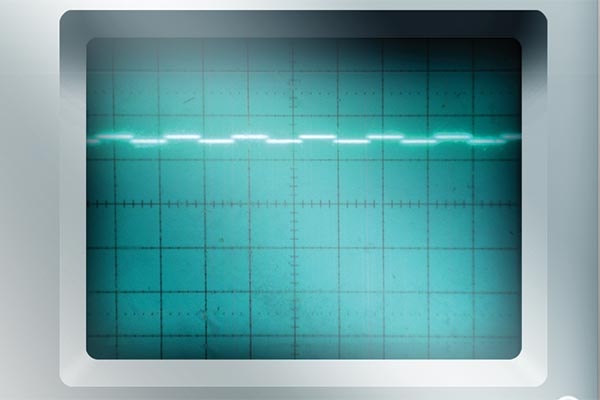
Photo 3. The oscilloscope displays something similar to a square wave. I have an oscillator circuit turning my small lightbulb on or off. The display shows the battery switching from open circuit to loaded, where the voltage swings from approximately 5.5 volts (no load) to 5.4 volts with a load.
Note: There are a couple of voltage dips down to 3.2 and 3.4 volts for roughly 1 millisecond (1/5 of a division or 1/1000 of a second). How low can the voltage go and still operate the radio system? Two systems must be powered in models—all of the servos and the receiver. If the voltage to the servos is decreased, we lose torque and speed. Many servos can function down to 3 volts, and the electronics in some servos can operate on slightly less voltage. The torque at this low voltage may be so low that when connected to a control surface, the surface does not move. When the voltage is restored, however, the servo quickly resumes normal operations. Our 2.4 GHz receivers have what is referred to as a brownout, in which a voltage drop may briefly turn the receiver off. When the voltage is restored, the receiver must relink.
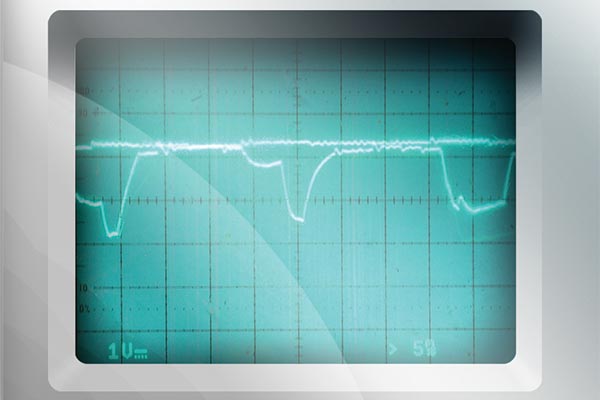
Photo 4. The oscilloscope is connected to the receiver bus displaying the voltage. The receiver battery is 4.8 volts and 500 mAh. There are four standard servos plugged into the receiver. The voltage drops as all of the servos operate back and forth. The left side of the screen shows a voltage dip to 3.2 volts and the center of the screen shows a dip to 3.4 volts.
Although the relink can be quick, we may feel it when flying. I have tested several receivers with a variable regulated power supply. In this test, I am only trying to determine where the receiver fails by observing its output on an oscilloscope. I slowly lowered the voltage until the receiver no longer operated. One brand dropped out at roughly 3.2 volts and many of the others dropped out at approximately 2.7-2.5 volts. A voltage drop that does not fall below 3.3 volts typically is not a problem. What about a higher-voltage battery? If a 4.8-volt battery is replaced with a 6-volt battery, the receiver will have a bigger cushion with which to work (4.8 volts down to 3.3 volts vs. 6 volts down to 3.3 volts). There also will be more torque and speed with the servos as long as they are designed to operate on the higher voltage. Keep in mind that when the voltage to any circuit is increased, the current also rises. The increase in current translates into shorter flight times and more heat (larger voltage drops) if there is resistance in the wiring or connectors. The biggest reason for voltage drops is resistance, which is in wires, connectors, switches, and even battery packs. The large voltage drops shown in the pictures were partly caused by a high-resistance cell in my old, 4.8-volt 500 mAh battery, yet the battery looks fine during a voltage check and when cycled. I typically would not see a voltage drop that low with a 500 mAh battery operating four servos, but four servos operating together is a large load and some drop in voltage will occur.
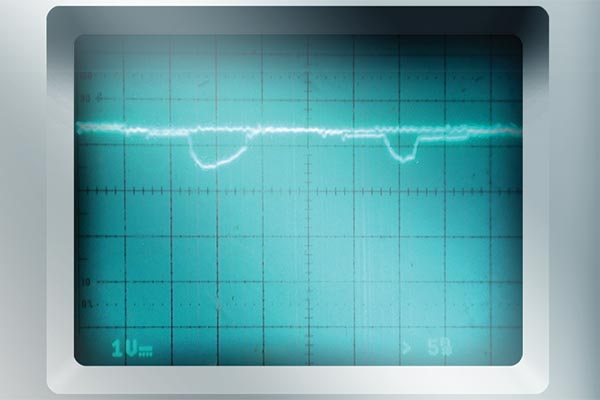
Photo 5. Photo 5 shows the same setup as Photo 4, except the battery was replaced with a 4.8-volt 2,700 mAh-capacity battery. The lowest voltage dip is approximately 4.5 volts.
When electronics fail, the failure is typically because of a mechanical connection, switch, connector, etc. Most of the time, our RC systems are powered by one battery, one switch harness, and one connector into the receiver—a failure here and we are finished. In many cases the battery connector is the same size as one servo connector, yet the battery connector has to feed all of the servos. If there is high resistance in the wiring, switching from 4.8 volts to 6.0 volts could make the voltage drop worse. Make sure that the switch harness is of good quality and that both poles of the switch are in parallel to make or break the connection to the red wire. The connectors need to have good connections and a tight fit—both the plastics and the pins. Your battery pack needs to be an adequate size and in good condition. If you are flying a larger airplane with more than five or six servos, you may want to use multiple battery connections to the receiver or even multiple battery packs.
Flying Electric
Many ESCs have a BEC that allows you to power your receiver or servos from the motor battery. This saves weight and makes installation and charging easier. Make sure to use a good-quality BEC and don’t exceed its current output with the size or number of servos. With larger models, many people opt to use a separate receiver battery instead of the BEC. You can use both. I prefer to use a BEC of 5.5 volts at a minimum of 4 amps. I then install a diode (of at least 4 amps) in the ESC’s servo connector (red wire) with the cathode toward the receiver. Because of the diode’s voltage drop, the receiver will receive slightly less than 5 volts from the BEC at the throttle port. I then install a five-cell (6-volt) battery with the normal switch harness plugged into the receiver battery port. My five-cell battery is the primary source of power for the receiver/servos because its voltage is higher than that from the BEC. If my 6-volt battery drops down, the BEC gradually steps in to help with the load. I figure that if I have a BEC onboard, I should put it to work, even if it is intended for backup power.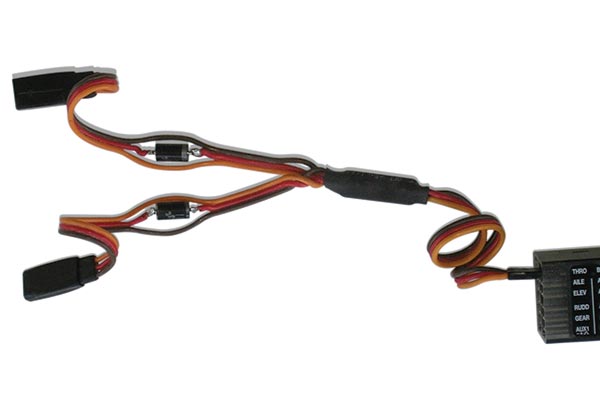
Photo 6. A Y harness with diodes plugged into the throttle port of a receiver. This Y harness would be used in a twin-engine electric aircraft with two ESCs. The two BECs would then act as backup power to our receiver and servos. Normally, the diodes would be insulated in heat-shrink tubing.
Are you flying an electric twin-engine airplane? You will have two ESCs and maybe two BECs. Both ESCs are typically plugged into a Y harness and then plugged into the receiver’s throttle port. In the throttle Y harness, add a diode (of at least 4 amps) to each leg of the Y in the red wire with the cathodes toward the receiver. The two diodes allow each BEC to power the receiver/servos without the two BECs fighting each other. The voltage into the receiver’s throttle port will be roughly 5 volts. The primary power to the receiver/servos is from the five-cell, 6-volt battery through a standard switch harness to the receiver’s battery port. Note: with either of these systems that I’ve discussed, the BEC used must be 5.5 volts and the primary battery must be a good five-cell, 6-volt battery. The servos must be designed to operate on six volts. —Cal Orr [email protected]
Comments
Good Article, I have thought
Good Article, I have thought about the same approach before but have never tried it.
Voltage vs current
The statement made is not true for constant power loads: "Keep in mind that when the voltage to any circuit is increased, the current also rises.". This can be seen via ohms law: VxA=W W is fixed
For a fixed load in watts, increasing the voltage decreases the amperage proportionately.
This is not a "constant power load"
The condition as described in the article is NOT always a constant Wattage or "power" situation.
In this case, a, voltage increase to the servos will, in turn, create an increase in current flow.
The increased voltage and the attendant current rise will create a larger power (Wattage) consumption.
When switching regulators are employed, the power level is, for the most part more constant. RC controls may have either or a mixture of both but generally speaking, increased voltage will cause an increase in Amperage.
Internal resistance/impedance of the cells we use...
One of the most-often ignored and/or forgotten parameters that a rechargeable cell is measured by is usually stated as its "internal resistance", or "internal impedance". Knowing the general R-int (just one abbreviation for this parameter) of the cells one is using in their battery packs is a CRITICAL figure to know, as to what sort of drain it can be placed under...and where it's best to use it in our models!
As I've built all my own radio gear for the hobby since 1977 - even the nickel-chemistry battery packs (first Ni-Cad in the late 1970s, changing over to NiMH cells around 2000) I've used for building all my own battery packs for powering the "knobby" single stick transmitters that I HAVE to use for the hobby (I canNOT safely fly with dual-stick radios), when I found out that the common AA-sized NiMH cells usually have a 25-30 milliohm R-int figure PER CELL in them...and then multiply that times four for a 4.8 VDC airborne pack, that adds up to as high as a 120 milliohm R-int figure. If one used something like those 30 millohm/cell NiMH cells in the rechargeable battery pack for something like a Spektrum receiver's airborne power source, "voltage dropouts" from such a high 120 milliohm value would be much more likely to cause a reboot, and a likely crash.
Conversely, if one was to use a LOW-impedance NiMH cell (usually a sub-C-size or some variant of that size format, like an 80%-lengthwise "4/5 Sub-C" sized cell) will give you an R-int figure of only some FIVE millliohms instead. Four such low-impedance NiMH cells would only add up to a 20 milliohm figure, a much more desirable choice for a sensitive receiver (sensitive to dropouts, that is) like a Spektrum, while the higher-impediance AA-sized cells can be much more appropriate for powering up a transmitter instead.
The best rechargeable cells available nowadays, A123's LFP (lithium iron phosphate, also abbreviated LiFePO4) cells have for their commonly used ANR26650 size (at 2.5 amp-hour capacity), a similarly "low" impedance around 5 to 6 milliohms apiece. Since only two are needed for most airborne needs (total of 6.6 volts), their series impedance of only some 12 milliohms at maximum can easily explain WHY they're so popular for airborne radio-hardware power needs. A trio of these same cells in series, adding up to 9.9 volts fully charged, can power many older radios and owner-built radios like my own "knobby boxes" can use, and with 2.5 amp-hours of power, one can fly nearly an entire weekend on just one charge, with only a 20 milliohm total impedance likely for three such cells in series.
Add new comment